By Namal Senanayake
September 2018
- Conquering stability and shelf-life issues of frying oils can be challenging. During deep-fat frying, several physical and chemical changes—mostly oxidation, polymerization, and hydrolysis—occur simultaneously, altering the chemical composition of edible oils during frying.
- Both synthetic and natural antioxidants are suitable for stabilizing frying oils against thermal lipid oxidation; however, in response to consumer demand for clean labels with no artificial ingredients, the food industry has been actively seeking sources of naturally derived antioxidants.
- Rosemary extract is one of the most important commercially available natural extracts, and is well-known for its positive attributes as a natural flavoring agent and source of natural antioxidants. This article highlights two case studies of the antioxidant activity of rosemary extract in canola frying oil.
Various types of edible oils are available for use in frying applications. These products range from plant oils, such as soybean, canola, sunflower, safflower, cottonseed, corn, groundnut, peanut, palm, palm kernel, coconut, and olive oils to animal fats, such as lard and tallow. Mid-oleic and high-oleic vegetable oils are exceptionally suitable for frying applications. These specialty oils can be used directly or blended with more readily available and cost-effective conventional vegetable oils. Considerations when selecting an edible fat or oil for frying include fatty acid composition, the type of commercial application, frying performance, the oil’s turnover rate, and the shelf-life of various fried foods. Numerous chemical changes can take place in edible oils and fats during processing, storage, and frying operations. The addition of antioxidants helps delay lipid oxidation and improves the shelf-life of edible oils and fats, including frying oils. Synthetic antioxidants are often added to edible oils and fats to retard oxidation during storage and frying; however, consumers increasingly view synthetic antioxidants as undesirable. Consequently, there is growing interest in naturally derived antioxidants for frying applications. This article provides an overview of the chemistry of frying, methods of measuring frying oil quality, and the action and fate of antioxidants during frying processes. Sources of natural antioxidants for frying oils are also discussed, with a primary focus on rosemary extract.
Chemistry of frying
The oxidation chemistry of edible oils and fats under frying conditions is extremely complex, involving both thermal and oxidation as well as hydrolysis reactions. When edible oil is exposed to atmospheric oxygen, moisture, and steam at elevated temperatures (typically 325–400oF) during frying conditions, a multitude of complex chemical reactions concurrently take place. These include hydrolysis, oxidation, polymerization, lipid decomposition, and other thermal reactions that result in the formation of numerous undesirable compounds. The hydrolysis reaction occurs when moisture from food is released into the heated oil. This produces steam, which causes the ester linkages of triacylglycerols to break down and form free fatty acids, monoacylglycerols, diacylglycerols, and glycerol. These breakdown compounds have higher polarities and lower molecular weights than the original unaltered triacylglycerols, and can further accelerate hydrolysis reactions in the oil. Meanwhile, the free fatty acids produced are rapidly oxidized and accelerate thermal oxidation by solubilizing transition metal ions in the oil. The free fatty acid content of frying oil typically increases as frying continues. In fact, extended frying increases the free fatty acid content so much that it can lower an oil’s smoke, flash, and fire points. As free fatty acids tend to be volatile, they can be lost through steam distillation. In addition, free fatty acids can degrade and produce other volatile compounds or react to form non-volatile components at elevated temperatures. Most reviews of the scientific literature indicate that oxidation and polymerization reactions are much more pronounced than hydrolytic reactions during deep-fat frying.
The reaction between atmospheric oxygen and oil causes thermal lipid oxidation. The chemical mechanism of thermal lipid oxidation is essentially the same as that of autoxidation and includes three basic steps: initiation, propagation, and termination; however, the rate of thermal oxidation is presumed to be faster than the free radical chain reactions that are associated with autoxidation. The main decomposition products of frying oil are complex mixtures of volatile monomeric compounds, non-volatile polar compounds, non-volatile triacylglycerol dimers and oligomers, and polar and non-polar polymeric substances. Volatile compounds produced by oxidative and thermal decomposition of lipids are saturated and unsaturated aldehydes, ketones, hydrocarbons, lactones, alcohols, acids, esters, furans, and aromatic compounds. These decomposition compounds affect the quality of the oil and will have an undesirable effect on the flavor and nutritional value of fried foods. The decomposition compounds accelerate further degradation of the oil, increase oil viscosity, reduce heat transfer, reduce oil smoke points, increase oil absorption in fried food, and lead to undesirable color in the frying medium and fried foods. The deep-fat frying process increases the degradation of unsaturated fatty acids, color, viscosity, foam development, free fatty acids content, polar compounds, and polymeric compounds. The main factors influencing the stability of oil during frying include the type of oil or fat used; the surface-to-volume ratio of the fryer; the type of fryer; frying temperature; frying time; the number of frying cycles; exposure to atmospheric air (oxygen), moisture, steam, and prooxidants; the presence or absence of antioxidants; and removal and replenishment of oil during frying.
Analytical assays for monitoring quality
There are many analytical methods available for assessing frying oil quality and antioxidant effectiveness during frying. These include physical tests that measure physical properties of the oil, chemical tests that are based on measurements of volatile and non-volatile decomposition products of oil, and instrumental methods that are available as rapid tests to assess frying oil deterioration by means of determining either volatile or non-volatile decomposition products of oil (Table 1). As many factors affect the rate of oil deterioration during frying, no single analytical protocol would be adequate under all frying conditions. Some analytical procedures are straightforward and simple, while others are rather complex. The methods that determine changes in physical properties of frying oil include measurements of color, viscosity, foam height, and smoke point.
TABLE 1. Laboratory methods of measuring frying oil quality and antioxidant effectiveness in frying oil |
Color |
Viscosity |
Foam height |
Smoke point |
Peroxide value |
Conjugated dienes |
p-Anisidine value |
Free fatty acids (%) or acid value |
Fatty acid composition |
Iodine value |
Dielectric constant |
Refractive index |
Total polar compounds (TPC) |
Dimerized and polymerized triglycerides |
Epoxy value |
Peroxide value (PV), a measure of hydroperoxides in an oil, is commonly used to assess the initial stages of oxidation. When unsaturated lipids undergo autoxidation, the main initial compounds formed are hydroperoxides. Hence, the PV is a measure of primary oxidation products of lipids. The PV is typically conducted using a titration-based method (AOCS Official Method Cd 8b-90) to determine the levels of iodine liberated from potassium iodide in an acid medium. Various other colorimetric methods are also available. The PV is usually reported in terms of milliequivalents of peroxide per kilogram of fat. Freshly produced refined, bleached, and deodorized (RBD) oils usually have a PV of well below 1.0 (meq/ kg). Oils and fats used for frying typically show a minor increase in PVs during frying. As lipid hydroperoxides decompose rapidly at elevated temperatures, the PVs of oils under frying conditions may be misleading. Hence, PV testing is not generally recommended for assessment of frying oil degradation; however, it may be used in some instances to compare the effectiveness of antioxidants during frying.
Conjugated dienes are also used to evaluate the initial oxidation of oils and fats, and they can be measured spectrophotometrically at 232 nm. This method is simple and involves dissolving a small amount of oil or fat in isooctane and measuring the absorbance using a UV spectrophotometer. Conjugated dienes initially increase as oil oxidizes; however, the amounts can decrease as the conjugated diene hydroperoxides react in further oxidative reactions. Because of conjugated diene hydroperoxides decomposition at elevated temperatures, a low level of conjugated dienes alone is not necessarily an indication of high quality, and measures of other parameters, including secondary oxidation products, may be necessary.
Another oxidation marker, the p-Anisidine test (AOCS Official Method Cd 18-90), measures the high-molecular-weight saturated and unsaturated carbonyl compounds in lipids. The p-Anisidine value is a measurement of the content of non-volatile aldehydes in an oil, primarily 2,4-dienals and 2-alkenals. Aldehydes, being secondary oxidation products produced during lipid oxidation, are the most likely compounds to generate unacceptable flavors and odors in fats and oils. In the presence of acetic acid, p-anisidine reacts with aldehydes in fats and oils to form products that absorb at 350 nm. The p-anisidine test is not suitable for measuring secondary oxidation in oils and fats that have a strong color or contain naturally occurring pigments, as these can interfere with the assay and yield invalid results.
The content of free fatty acids (AOCS Official Method Ca 5a-40) has historically been measured during frying operations as an indicator of deterioration in frying oil. Free-fatty-acid analysis is used to determine the degree of hydrolysis. Free fatty acids (FFAs) are volatile and evaporate rapidly during frying, so these compounds may not be the best quality indicator. In an investigation at the Camlin Fine Sciences laboratories, 14 canola oil samples with varying FFA contents were collected from an industrial-scale fryer. Fresh oil samples were also obtained for the analyses, which included the determination of FFAs, Oil Stability Index (OSI), and p-anisidine values. The samples evaluated had FFAs ranging from 0.02 to 4.1%. A wide variation in p-anisidine values for oil samples—from 4.1 to 71.0—was also encountered, indicating that most oil samples were highly oxidized. A correlation analysis exploring the relationships between FFAs and p-anisidine values or OSI values was performed. Poor correlations were found between FFAs and p-anisidine values and between FFAs and OSI values. Hence, it was construed that FFA content was an inaccurate indicator of heat damage in frying oils.
Oil viscosity, being one of the most important physical oil properties, can be used to assess frying oil quality. Typically, the viscosity of oil increases with frying time. An oil’s absolute viscosity can be measured using a conventional viscometer, which measures the torque required to rotate an object, such as a spindle, in an oil. In this test method, the oil is placed in a glass beaker and maintained at a steady temperature. A spindle is then rotated in the oil at a fixed speed, and the torque required to turn the spindle is measured. The internal resistance to rotation contributed by the shear stress of the oil is then used to determine the absolute viscosity of the oil.
The measurement of total polar compounds (TPC) is one of the most popular methods used to assess the cumulative degradation of frying oils. The TPC (AOCS Official Method Cd 20-91) is defined as the sum of all compounds in an oil except for the original triacylglycerols. These compounds include oxidized monomers, dimerized triacylglycerols, polymerized triacylglycerols, monoacylglycerols, diacylglycerols, and free fatty acids, along with other oil-soluble constituents that are more polar than the original triacylglycerols. In Europe, the measurement of TPC has been accepted as a standard reference protocol to assess the quality of fats and oils during extreme frying conditions. As TPC is one of the best indicators of frying oil quality, several European countries have established regulatory limits of 25–27% (w/w) TPC for deteriorated frying oils; however, no regulations have been established in the United States.
Determination of dimeric and polymeric triacylglycerols (DPTG) is also widely used to assess frying oil degradation, and it is a routine regulatory protocol in some European countries. The development of polymeric compounds is one of the most notable changes in edible oils and fats during frying. High-performance, size-exclusion chromatography (HPSEC) appears to be a standard method for the determination of polymerized triacylglycerols. Regulations in some European countries specify that frying oil should contain no more than 10–16% DPTG, a level when deterioration is objectionable and the oil should be discarded.
Antioxidants
Antioxidants are typically used to enhance shelf life and preserve the quality of edible oils and fats. They suppress oxidation reactions by participating in or interfering with the lipid autoxidation reaction cascade through various mechanisms. Antioxidants used in oils and fats must be cost-effective, safe, easy to handle and use, readily available, stable, effective at low concentrations, and devoid of any undesirable flavor, odor, or color attributes. The antioxidants for frying oils should remain stable when exposed to frying temperatures and also provide protection to the foods fried in these oils, thus increasing the shelf life of the end products. They are typically added to frying oils early in their production to protect the oils during handling, transport, and storage.
Antioxidants can be categorized according to their mechanism of action. Primary antioxidants are free radical scavengers that delay the initiation stage or interrupt the propagation step of autoxidation. Synthetic antioxidants such as butylated hydroxyanisole (BHA), butylated hydroxytoluene (BHT), propyl gallate, and tertiary butylhydroquinone (TBHQ) are well-known, potent primary antioxidants. Various regulatory organizations, including US Food and Drug Administration (FDA), have placed tolerance limits on the amounts of synthetic antioxidants that can be added to fats and oils (typically below 200 ppm). Secondary antioxidants slow down the rate of oxidation reactions by functioning as chelators for prooxidant metal ions, providing hydrogen to primary antioxidants, decomposing hydroperoxides to non-radical species, deactivating singlet oxygen, absorbing ultraviolet radiation, or acting as oxygen scavengers and enhancing antioxidant activity of primary antioxidants. Examples of important secondary antioxidants include ascorbic acid, ascorbyl palmitate, carotenoids, and citric acid, among others. Some antioxidants exhibit primary and secondary mechanisms of activity.
Stabilizing edible oils and fats under frying conditions can be challenging because the rate of oxidation under extreme conditions of temperature and time is quite high, and poor thermal stability of some antioxidants may cause premature decomposition. Furthermore, most antioxidants tend to be somewhat volatile and suffer evaporative losses during frying. Antioxidants are not only consumed rapidly during free-radical oxidation, but they also degrade at elevated temperatures during frying (Fig. 1). Thus, the desired level of antioxidants is not sustained during frying operations. TBHQ is a synthetic phenolic antioxidant that has been widely used in the food industry to improve the stability of edible oils during frying. TBHQ is relatively stable at high temperatures and less volatile than BHA or BHT. The protective effects of TBHQ not only improve the stability of oils during frying, but also carry through to the fried foods, thereby extending their shelf life. Food-grade methyl silicone or dimethyl polysiloxane are also typically added to frying oils to help inhibit foam formation and extend fry life by retarding thermal oxidation. Silicones are effective polymerization inhibitors. They increase the smoke point of oils—possibly by providing a surface-to-air barrier. There is also evidence that antioxidants and silicones exhibit synergistic effects. Consequently, both to frying oils may improve quality more than TBHQ or silicones alone.
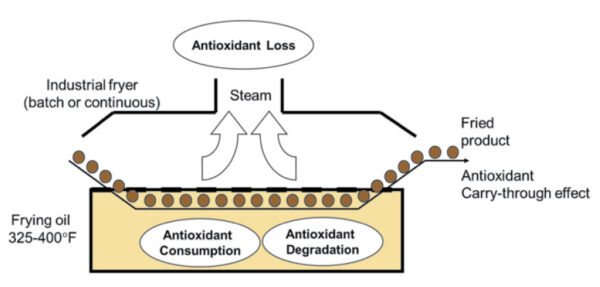
FIG. 1. Heat stability and volatility of antioxidants during frying.
Plant-based antioxidants typically offer natural and safer alternatives to some of the synthetic antioxidants commonly used in frying oils. A plethora of scientific studies published to date have reported the behavior of natural antioxidants under frying conditions. Some of the antioxidants that have been studied include the tocopherols; tocotrienols; squalene; phytosterols; phospholipids; corn-oil or rice bran oil-derived steryl ferrulates; sesame oil lignans such as sesamol, sesamin, and sesamolin; and various natural extracts derived from rosemary, oregano, grape seed, sage, thyme, pomegranate, olive, citrus peel, and green tea, among many others.
Tocopherols, the most widely dispersed antioxidants in nature, represent the primary antioxidants in vegetable oils. Tocopherols exert their utmost antioxidant activity when they are present at relatively low levels in vegetable oils. At very high concentrations, they may behave as pro-oxidants. As free-radical scavengers, tocopherols play a significant role in protecting vegetable oils from oxidative degradation during frying. However, the efficacy of natural tocopherols that have been added to oils during the frying process is debatable. Meanwhile, although numerous natural antioxidants have been researched and reported, only a handful of naturally derived antioxidants are commercially available for use in frying oils. These include rosemary extract, mixed tocopherols, sage extract, and green tea catechins, along with some other additional ingredients that exhibit antioxidant properties or function as synergists.
Rosemary extract
Rosemary (Rosmarinus officinalis, L) is an aromatic perennial herb which belongs to the family of Lamiaceae. Native to the Mediterranean region, the herb has a long history of safe use as a food flavoring. Its extract contains several active compounds that have been shown to exert antioxidant functions that slow down the oxidation of unsaturated lipids in food. These active antioxidant molecules belong primarily to the classes of phenolic acids, phenolic diterpenes, and triterpenes. The principal antioxidant components of the leaf extracts are lipid soluble, notably carnosic acid and its primary breakdown component carnosol, collectively known as the phenolic diterpenes. The carnosic acid molecule possesses three six-membered rings. including a dihydric phenolic ring, and a single free carboxylic acid group. Carnosol, the main oxidized derivative of carnosic acid, also has a molecular structure consisting of three six-membered rings that include a single aromatic ring with two hydroxyl groups and a lactone ring (Fig. 2). More than 90% of the antioxidant activity of rosemary extract is attributed to the presence of carnosic acid and carnosol. Among phenolic diterpenes, carnosic acid is the major compound present in rosemary extract, along with smaller quantity of carnosol. Hence, the antioxidative response of rosemary extract is largely due to the greater abundance of carnosic acid present in the extract. Both diterpenes can be further oxidized, on exposure to elevated temperatures and light, into products that remain active as antioxidants. Hence, their ability to control oxidation is maintained. Carnosic acid and carnosol are particularly effective antioxidants for frying oils at elevated temperatures, and their antioxidant activities carry over into the fried goods. It has been suggested that the antioxidant activities of carnosic acid and carnosol follow a mechanism equivalent to that of other phenolic antioxidants—a mechanism that is attributed to the presence of two ortho-phenolic OH groups located at C11 and C12 positions of catechol moieties that can serve as proton donors to lipid free radicals.
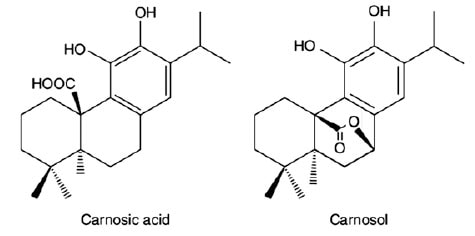
FIG. 2. Phenolic diterpenes present in rosemary extract.
Commercial extracts of rosemary are typically produced through solvent extraction of the dried rosemary leaves. Food-grade ethanol, hexane (used separately or in combination), acetone, or supercritical CO2 may be used as extraction solvents. The solvents are subsequently removed using heat and vacuum. Other processing steps may include filtration, purification, drying, and sieving. The resulting extracts are subsequently deodorized, decolorized, and standardized using food-grade diluents and carriers. Commercial rosemary extracts are offered as powders or liquids. Liquid extracts are available in several forms depending on their solubility characteristics: oil-soluble, oil-dispersible, water-soluble, and water-dispersible. Among these, oil-soluble and oil-dispersible liquid extracts are typically produced by rosemary extracts containing nonpolar active compounds along with vegetable oils or propylene glycol as carriers. Meanwhile, water-dispersible liquid extracts are based on nonpolar active components of rosemary extract with some emulsifiers and carriers. On the other hand, rosemary extracts containing polar active components along with liquid carriers are used to produce water-soluble liquid extracts. As for powdered extracts, they can be either oil-dispersible or water-dispersible.
Rosemary extract has conventional worldwide approval and is traditionally positioned as a flavoring agent for use mostly in food. However, local food regulations should always be consulted, as the specific regulatory requirements regarding its intended use, safety and technical suitability in food may differ from one country to the next. In the United States, rosemary extract is Generally Recognized as Safe (GRAS) for use in foods as a natural flavoring when used in accordance with 21 CFR 182.10 and 21 CFR 101.22. It may be declared on food labels as rosemary extract, natural spice extract, or natural flavoring. As rosemary extract demonstrates both antioxidant and flavoring properties, both functions may be utilized within a food. Rosemary extract, produced using one of the four solvent extraction techniques (by means of acetone, ethanol, or two-step extraction using hexane and ethanol, or supercritical CO2), has been approved by the European Union (EU) under Directives 2010/67/ EU and 2010/69/EU, and has been added to the official list of acceptable food additives for use in food. Accordingly, if rosemary extracts are added to food primarily as an antioxidant, specifications laid down in the EU Directives must be met. Consequently, food manufacturing companies can elect to label it as “Antioxidant: Extract of Rosemary” or with the E Number E-392. However, the definition of natural flavorings, as stated in EU Council Directive 88/388/EEC, still applies to certain types of rosemary extracts when used as flavorings, and they can be used in food applications unless legislations confine the use of flavorings in some specific foods.
Case study 1
In a recent frying oil study conducted by Camlin Fine Sciences laboratories, the efficacy of rosemary extract in canola oil was compared against a control oil sample with no additives. Commercially available rosemary extract liquid (an oil-soluble form) was added to canola oil and mixed well prior to heating. The oil samples were then heated to 400oF in a bench top fryer. Once the oil reached 400oF, 100-gram samples of french-fry-cut potatoes were fried for 5 minutes. Six frying cycles were completed consecutively with new fries for each cycle. Once all the frying cycles were completed, the oil was held for 4 hours at 400oF. Oil samples at various stages of frying and heating were collected for analytical testing. Conjugated dienes—the levels of primary oxidation products—in both samples increased with frying/heating time; however, the oil sample treated with rosemary extract had lower levels of conjugated dienes as compared to the negative control sample (Fig. 3).
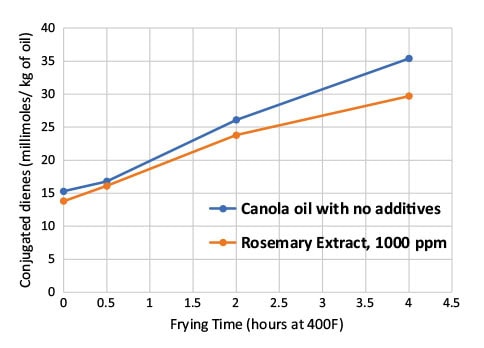
FIG. 3. Conjugated dienes of canola oil with and without rosemary extract during frying.
Anisidine values, a measurement of the secondary oxidation products of lipids, indicated that the rosemary extract-supplemented oil sample had lower levels of anisidine values during frying/heating than the control oil sample (Fig. 4). This indicates that oxidative stability of the oil improved by adding rosemary extract.
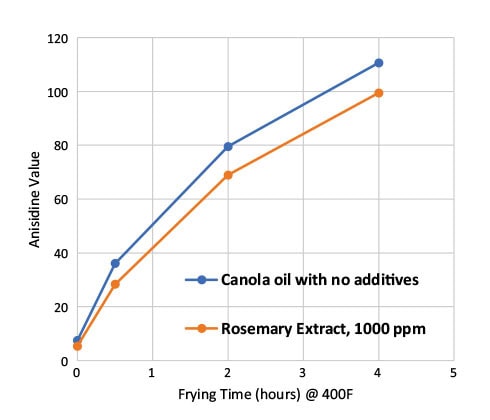
FIG. 4. Anisidine values of canola oil with and without rosemary extract during frying.
The Oil Stability Index (OSI) of samples collected during various stages of frying were measured with the Oxidative Stability Instrument according to AOCS Official Method Cd 12 b-92. The OSI values of oil samples decreased during frying and heating, with the rosemary extract-treated oil sample remaining significantly higher than the control oil sample (Fig. 5). These data suggest that rosemary extract-treated oil sample had a longer “fry-life” than the control oil with no additives.
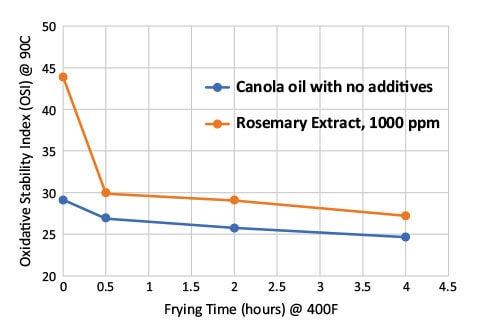
FIG. 5. Oil Stability Index (OSI) of canola oil with and without rosemary extract during frying.
The viscosity of oil samples increased continually with the number of frying cycles and heating time (Fig. 6), suggesting that viscosity may be used as a parameter to indicate vegetable oil degradation. The rosemary extract-treated oil sample had lower viscosity values during frying/heating as compared to the control oil sample, indicating that the oxidative stability of the oils was improved by adding rosemary extract.
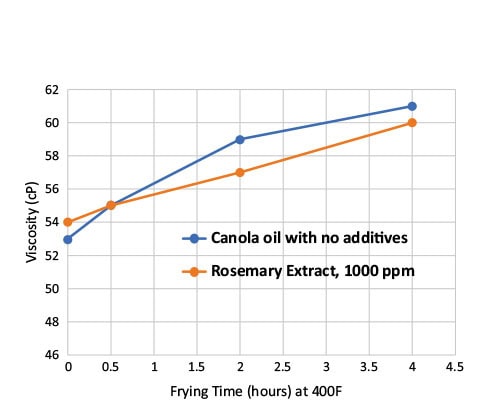
FIG. 6. Viscosity values of canola oil with and without rosemary extract during frying.
Case study 2
In another study conducted by Camlin Fine Sciences laboratories, the efficacy of rosemary extract was compared with that of TBHQ. This study compared the antioxidant capacity obtained from 1000 ppm rosemary extract with that obtained from 200 ppm TBHQ using canola oil as the frying medium. The canola oil with no additives served as the negative control. The oil samples were heated to 400oF in a bench top fryer. Once the oil is at 400oF, french-fry-cut potatoes were fried for 2 minutes and 30 seconds. For each treatment, 40 frying cycles were completed consecutively with new fries for each cycle. Oil samples at various stages of frying were collected for analytical testing.
Peroxide values and anisidine values were measured during frying (Tables 2 and 3). Oil samples prior to frying had peroxide value of less than 1.0 (meq/ kg), which is below the manufacturer’s specification. At the end of 40 frying cycles, the control sample with no additives had the highest peroxide value followed by TBHQ and rosemary extract-supplemented oils (Table 2). Anisidine values of oil samples increased with the number of frying cycles for all three oils. Based on anisidine values, the oxidative stability of oil samples was: rosemary extract > TBHQ > negative control with no additives (Table 3).
TABLE 2. Laboratory methods of measuring frying oil quality and antioxidant effectiveness in frying oil Sample Percent (%) Increase in peroxide values after 40 frying cycles Canola oil with no additives 88.5 TBHQ, 200 ppm 17.2 Rosemary extract, 1000 ppm 11.9
TABLE 3. Anisidine values of frying oils p-Anisidine value Before frying After 10th frying
After 20th frying After 30th frying After 40th frying Canola oil with no additives 1.1 23.7 36.7 45.5 54.2 TBHQ, 200 ppm 1.3 21.6 34.0 44.6 53.4 Rosemary extract, 1000 ppm 0.9 17.8 34.2 40.2 46.2
The oil sample treated with TBHQ had the highest OSI values followed by rosemary extract and negative control (Fig. 7). Based on the OSI test results, the oxidative stability of oil samples was: TBHQ > rosemary extract > negative control with no additives.
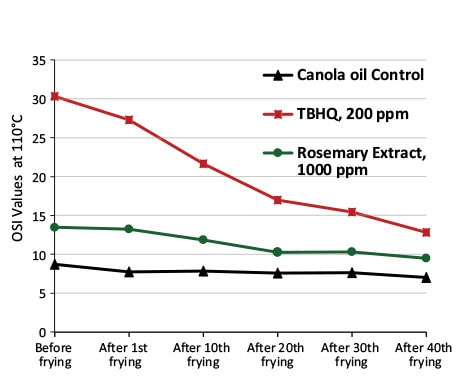
FIG. 7. Oil Stability Index (OSI) of frying oils.
Namal Senanayake is a scientific manager at Camlin Fine Sciences North America, a subsidiary of the parent company Camlin Fine Sciences Limited in Mumbai, India. He has over 20 years of experience in lipid chemistry, lipid oxidation, and antioxidants, and has held various roles in food industry and academic institutions. At Camlin Fine Sciences, he is focused on managing quality control testing of antioxidant products; application testing of human food, pet food and animal feed products; developing new antioxidant products; and optimizing existing product formulations. He can be reached at namal.senanayake@camlinfs.com.
Further reading
- Hwang, H.-S. and Winkler-Moser, J.K. (2016). “Oxidative stability and shelf-life of frying oils and fried foods.” In: Hu. M., Jacobsen, C. (Eds.). Oxidative Stability and Shelf Life of Foods Containing Oils and Fats. Academic Press and AOCS Press, 251–285.
- Choe, E. and D.B. Min, Chemistry of deep-fat frying oils, J. Food Sci. 72: R77–R86, 2007.
- Senanayake, S.P.J.N. (2013). Rosemary and green tea extracts as natural antioxidants: Chemistry, technology and applications. In: Logan, A., Nienaber, U., Pan, X. (Eds.). Lipid Oxidation: Challenges in Food Systems, AOCS Press, 417–438.
Related Resources
Lipid Library
Edible Oil Processing
In the present context, the term edible oil processing covers the range of industrial…
Lipid Library
The Highs and Lows of Cannabis Testing
October 2016 With increasing legalization of both adult recreational and medical cannabis,…
Lipid Library
The secrets of Belgian chocolate
By Laura Cassiday May 2012 Like a bonbon nestled snugly in a…