Developments in green surfactants for enhanced oil recovery
By Raj Shah and John Calderon
March 2021
- Scientists are developing improved surfactants for enhanced oil extraction that have superior capabilities while being environmentally friendly and capable of strong operational tolerances to pH, salinity, and temperature.
- In laboratory tests, numerous green surfactants synthesized from vegetable oils and other plant-based materials matched or exceeded the capabilities of conventional synthetic surfactants.
- Plant-based zwitterionic surfactants are reported to have strong interfacial reduction values and operational tolerances.
More Informed Poster Summary
Download a PDF poster of this topic with additional figures and graphics.
This poster is part of a new digital platform from AOCS called More Informed. Visit the More Informed page to discover other opportunities to continue learning beyond the pages of the magazine.
Despite the continuing development of sustainable sources of energy, crude oil and natural gas resources remain crucial elements of the international economy. The oil and natural gas exploration industry alone is worth $86 trillion and represents 3.8% of the global economy [1]. With global petroleum and liquid fuel demand continually increasing and production set to reach 99.71 million barrels per day by 2021, improving the efficiency of extraction from existing natural reserves of petroleum is of utmost importance as the world gradually transitions away from fossil fuels toward more sustainable sources [2]. Toward that end, enhanced oil recovery (EOR) techniques have been developed and are used to minimize the amount of crude oil and petroleum that is left behind in underground reservoirs from conventional drilling extraction methods.
EOR, also known as tertiary oil recovery, follows the primary and secondary production stages of conventional oil extraction. These first two stages use the initial pressure energy stored in the reservoir to extract the oil (primary), then water or specialized gases to maintain pressure for a secondary extraction (secondary), ultimately yielding a total oil recovery of 40–60% [3]. A significant amount of remaining oil is trapped within the reservoir due to capillary forces that can vary depending on the type of rock structure, porosity, temperature, and other factors. EOR is used to overcome these capillary forces and increase recovery yields through a multitude of methods which can generally be classified into thermal, non-thermal, and microbial techniques [3,4]. The most commonly used and well understood EOR technique is thermal EOR (TEOR).
Chemical EOR (CEOR) techniques, used since the 1980s, are non-thermal EOR techniques that use the injection of water-soluble chemical agents, such as polymers, surfactants, alkalis, or a mixture of all three (Fig. 1). These agents can be mixed with water used during the secondary stage of extraction or as an additional tertiary stage, with each type of chemical agent improving net oil recovery through different mechanisms. Polymers increase the viscosity of the aqueous phase, thereby improving the ability of the solution to push oil out of pores on a macroscopic scale. Surfactants reduce the interfacial tension (IFT) between the oil and water solution, allowing greater microscopic displacement of oil through the formation of oil-water emulsions. Finally, alkalis react with acidic components in crude oil to form natural surfactants that reduce IFT in the same manner as synthetic surfactants [7,8]. These agents can be used individually or in combination, depending on geological and economic factors. Multiple CEOR projects have been successfully established internationally, but today CEOR is limited by high upfront capital and material costs, loss of surfactant/polymer due to adhesion to reservoir rock beds, and significant concern over the environmental impact of the chemicals that are used.
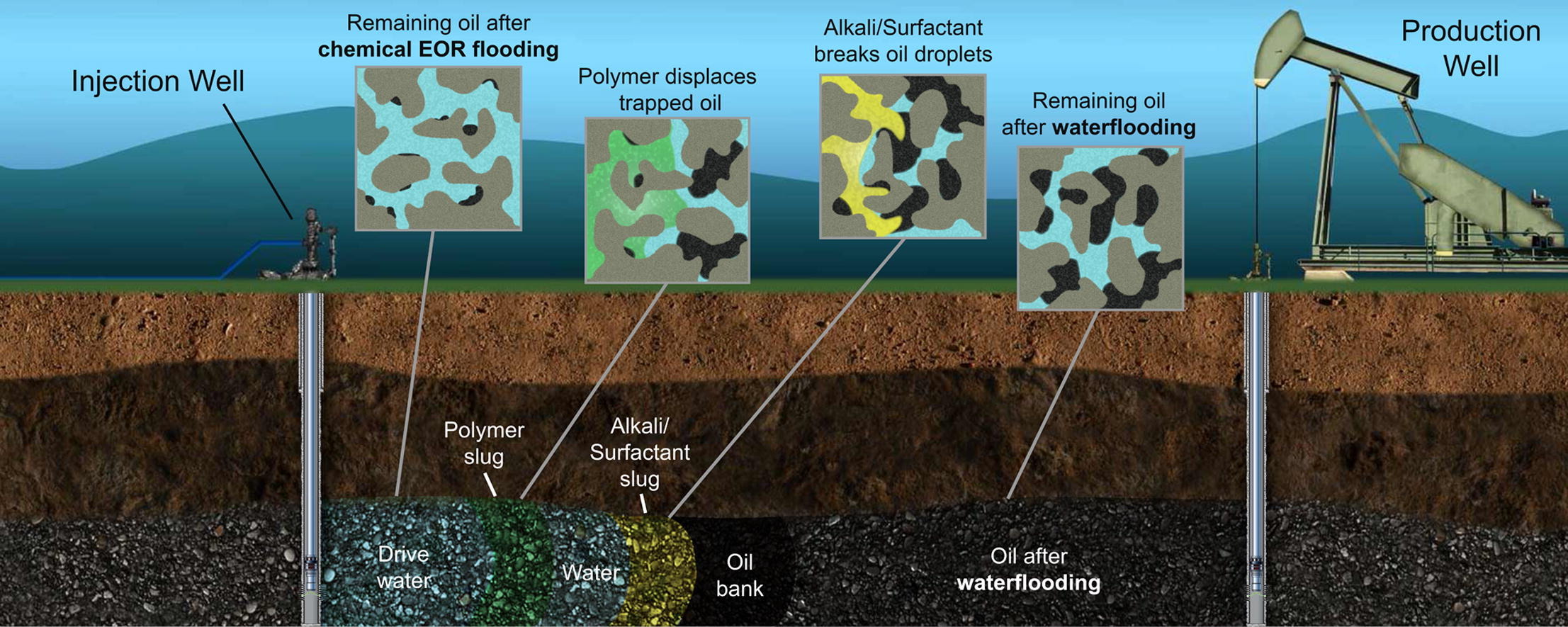
In the United States, EOR well operations are monitored and regulated by the US Environmental Protection Agency (EPA) through the Underground Injection Control Program (UIC), established in 1980 as a way to safeguard underground drinking water sources protected under the Federal Safe Drinking Water Act passed in 1974. All EOR operations fall under class II well designations for oil and gas recovery under the UIC and must meet minimum requirements to obtain and keep permits for well operation [4]. With oil demand continuing to rise and oil reservoirs maturing over time, the use of CEOR is expected to expand, and with it, the importance of using effective surfactants that are as environmentally friendly as possible.
How CEOR surfactants work
CEOR surfactants reduce interfacial tension (IFT) between the oil and water solution to enable greater mobility. This interaction arises from the amphiphilic nature of surfactants, which allows them to be soluble in both water and organic solvents [7]. Surfactants have a hydrophilic head and hydrophobic tail that work together to adsorb onto the oil/water interface. This reduces IFT and weakens the capillary forces trapping the oil within rock pores. A separate interaction known as wettability alteration can occur simultaneously and further improve oil recovery. Wettability alteration alters the contact angle of oil on the rock surface from an “oil-wet” state (contact angle θ > 90°) to a “water-wet” state ( θ < 90°) through desorption caused by the surfactant. The end result is a similar weakening of the capillary forces holding the oil in place and a corresponding increase in oil recovery. A visual representation of both mechanisms on an oil reservoir is depicted in Figure 2 [10].
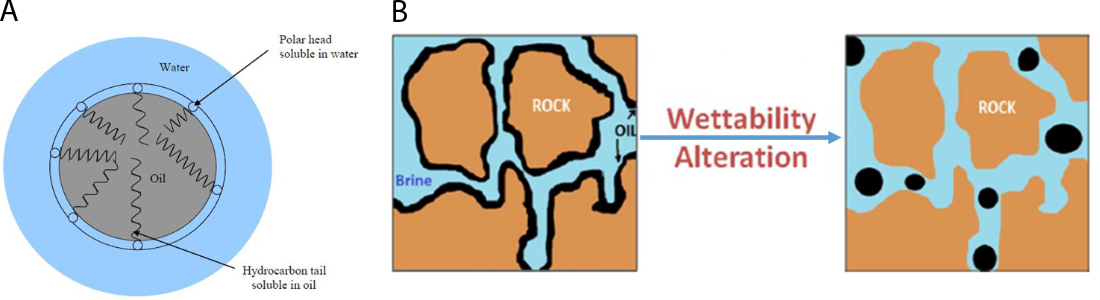
The surfactants used to activate these mechanisms can be classified into four major classes: anionic, cationic, non-ionic, and zwitterionic. These classes refer to the charge on the hydrophilic head, with anionic surfactants having a negative charge, cationic having a positive charge, non-ionic having no charge, and zwitterionic having both a negative and positive charge. Each type of surfactant has its own advantages and disadvantages. Anionic surfactants are the most commonly used due to their effective wettability alteration and IFT reduction. Cationic surfactants are particularly effective for carbonate rocks and clays but are more expensive than anionic surfactants. They are also unsuitable for sandstone due to strong adsorption onto the rock surface caused by the opposite charge of the rock. Non-ionic surfactants exhibit high tolerance to salinity and hardness but are less effective for IFT reduction. Zwitterionic surfactants, which are relatively new and less explored, combine the useful properties of anionic and cationic surfactants with high temperature, salt, and pH tolerance [7,10].
As a result of these inherent advantages and disadvantages, the operational conditions of the reservoir (rock type, salinity, temperature, etc.) have a significant impact on the type of surfactant used and, thus, the chemical structure of the surfactant. In general, carboxylates, sulphates, or sulphonates produce the surface-active negatively charged head in anionic surfactants, while non-ionic surfactants tend to utilize alcohols, such as the NEODOL compound and its derivatives developed by Shell researchers [11]. Cationic surfactants include bromide and chloride derivatives, while past investigations have used betaines to create the less-explored zwitterionic surfactants [10,11]. Choosing specific chemical groups can further alter properties, such as salinity and temperature resistance as well as compatibility with other chemicals, as occurs in Surfactant-Polymer (SP) or Alkali-Surfactant-Polymer (ASP) wells. Several examples of surfactants that have been investigated/used of each type alongside their chemical structures are shown in Table 1 [10].
![TABLE 1. Examples of previously investigated surfactants and their corresponding chemical structure for each type of surfactant [10]](/images/inform/table1.jpg)
Green surfactants
Although current synthetic surfactants provide an excellent range of capabilities for IFT reduction, wettability alteration, and operational tolerances, the cost-to-performance scalability for oil well operations is still too small for practical applications outside of mature and/or thin pay zone oil reservoirs. Adsorption of the surfactant onto the rock material results in loss of active surfactant and increases costs. These challenges, along with growing environmental concerns, signal the need to develop surfactants with superior IFT reduction and wettability alteration that are also environmentally friendly and non-toxic. Early investigations by Saeed Majidaeie, et al., in 2011, found that Methyl Ester Sulfonates (MES) synthesized using Jatropha curcas oil as feedstock were capable of reducing the IFT of a solution of itself and oil from the Dulang oilfield to 0.078 mN/m with a concentration of 0.25% by weight. This is an acceptable concentration for surfactant CEOR operations and illustrates the potential of using vegetable oils as alternative feedstocks [12]. Since then, numerous investigations of green surfactants developed from vegetable oils and other natural sources have observed strong IFT reduction, wettability alteration, and operational tolerance performances.
In nearly every case, these investigations take advantage of the unsaturated fatty acids or lignin that constitute many vegetables and plant sources to synthesize an effective surfactant for CEOR. With regard to surfactant adsorption, in 2016, Ademola M. Rabiu, Samya Elias, and Oluwaseun Oyekola synthesized a sodium epoxidized methyl ester sulfonate (SEMES) from waste vegetable oil and compared its performance to common commercially used synthetic surfactants like sodium dodecylsulfate (SDS) and cetyltrimethyl-ammonium bromide (CTAB) across several rock types: kaolin clay, ilmenite, silica, and alumina. Adsorption measurements in terms of adsorption density (mg of surfactant/g of adsorbent) found that SEMES showed lower adsorption density compared to SDS (an anionic surfactant) and CTAB (a cationic surfactant) on kaolin clay and ilmenite absorbents, shows the adsorption densities across a range of initial concentrations (Fig. 3), indicating the strong oil recovery efficiency of the SEMES surfactant and subsequent potential for lower costs [13].

Investigations into other plant-based sources have yielded similar promising results in a multitude of other areas as well. A surfactant synthesized from oil palm empty fruit bunches showed sufficiently low IFT of 0.204 mN/M with 1% concentration and strong thermal stability across 3 months. IFT values ranged between 0.204 mN/M and 0.125 mN/M with clear froth formation for oil separation [14]. An oil recovery study performed by Kevin Woe, et al., using a non-ionic surfactant also derived from palm oil found oil recovery values of up to 78.07% and IFT values as low as 2.08 x 10-3 dyne/cm; a spontaneous imbibition test at a reservoir temperature of 60°C and a concentration of 0.3% showed strong oil recovery potential at optimal conditions [15]. Perhaps one of the most promising endeavors comes from a zwitterionic surfactant dubbed CPDB synthesized from castor oil by Zhang, et al., in 2015 [16]. IFT measurements were performed against crude oil derived from the Daqing oil field at a range of pH (3–12) and salinity (5,318–12,223 mg/L) conditions to simulate performance against Shengli, Xinjiang, and Huabei crude oils. The synthesized surfactant achieved an extremely low interfacial tension of 5.3 x 10-3 mN/m against Daqing crude oil without any extra alkali at a concentration of 0.010 g/L while maintaining strong thermal stability and salinity/pH resistance [16]. Such strong performance merits further exploration of the capability of naturally derived zwitterionic surfactants and other castor oil surfactants.
As demand for oil continues to grow alongside energy demands, many maturing oil fields will require enhanced oil recovery techniques like CEOR to adequately provide stable supply. Countries with mature oil fields, such as the United States and Singapore, have invested efforts into developing improved surfactants. Continued development of green surfactants for CEOR will achieve greater yields with minimal environmental harm to support the world economy as it transitions to alternative energy sources for a more sustainable future.
About the Authors
Raj Shah is a Director at Koehler Instrument Company, New York, and an active AOCS member for the last 25 years. A PhD graduate in chemical engineering from Penn State university and a Fellow from The Chartered Management Institute, London, Shah recently co-edited a reference bestseller titled, “Fuels and lubricants handbook,” published by ASTM (https://tinyurl.com/y32djfza). He is an elected fellow at Energy Institute, NLGI, STLE, IChemE, INSTMC, AIC, CMI, and RSC, and a Chartered Petroleum Engineer. A recently elected Fellow by the Institution of Chemical engineers, UK, Shah was also honored with an esteemed engineer designation by Tau Beta Pi, the highest engineering honor society in USA. More information on Raj can be found at https://www.che.psu.edu/news-archive/2018/Alumni-Spotlight-Raj-Shah.aspx.
John Calderon is a chemical engineering student from Stony Brook University, where Shah is the Chair of the external advisory board of directors. John is also part of a thriving internship program at Koehler instrument Company.
References
[1] https://tinyurl.com/yyeprjm4
[2] https://tinyurl.com/y99s9mse
[3] Bachari, Zahra, et al. Application of natural surfactants for enhanced oil recovery – critical review., IOP Conference Series: Earth and Environmental Science 221: 012039, 2019, doi:10.1088/1755-1315/221/1/012039.
[4] Gbadamosi, A.O., R. Junin, and M.A. Manan, et al., An overview of chemical enhanced oil recovery: recent advances and prospects, Int. Nano. Lett. 9: 171–202, 2019, https://doi.org/10.1007/s40089-019-0272-8
[5] https://tinyurl.com/yxa7mmdf
[6] https://tinyurl.com/yyyon38k
[7] https://tinyurl.com/y3ey9np5
[8] https://tinyurl.com/y68cbt77
[9] Negin, C., S. Ali, and Q. Xie, Most common surfactants employed in chemical enhanced oil recovery, Petroleum 3: 197–211, 2017,https://doi.org/10.1016/j.petlm.2016.11.007
[10] Majidaie, S., M. Muhammad, I.M. Tan, and B. Demiral, Green surfactant for enhanced oil recovery, 2011 National Postgraduate Conference, Kuala Lumpur, 2011, 1–5, doi: 10.1109/NatPC.2011.6136533.
[11] Rabiu, A.M., S. Elias, and O. Oyekola, Evaluation of surfactant synthesized from waste vegetable oil to enhance oil recovery from petroleum reservoirs, Energ. Procedia 100: 188–192, 2016, https://doi.org/10.1016/j.egypro.2016.10.163
[12] Prakoso, N.I. and S. Purwono, Synthesis and application of green surfactant from oil palm empty fruit bunches’s lignin for enhanced oil recovery studies, chemical engineering transactions 63: 739–744, 2018, doi:10.3303/CET1863124.
[13] Kevin Woe, R. Setiati, and Y.F. Alli, A laboratory study of chemical enhanced oil recovery (CEOR) by spontaneous imbibition of non-ionic palm-oil based surfactant solution, AIP Conference Proceedings 2223, 040009 (2020); https://doi.org/10.1063/5.0006102
[14] Zhang, Q.-Q., B.-X. Cai, and W.-J. Xu, et al., Novel zwitterionic surfactant derived from castor oil and its performance evaluation for oil recovery, Colloids and Surfaces A: Physicochemical and Engineering Aspects 483: 87–95, 2015,https://doi.org/10.1016/j.colsurfa.2015.05.060